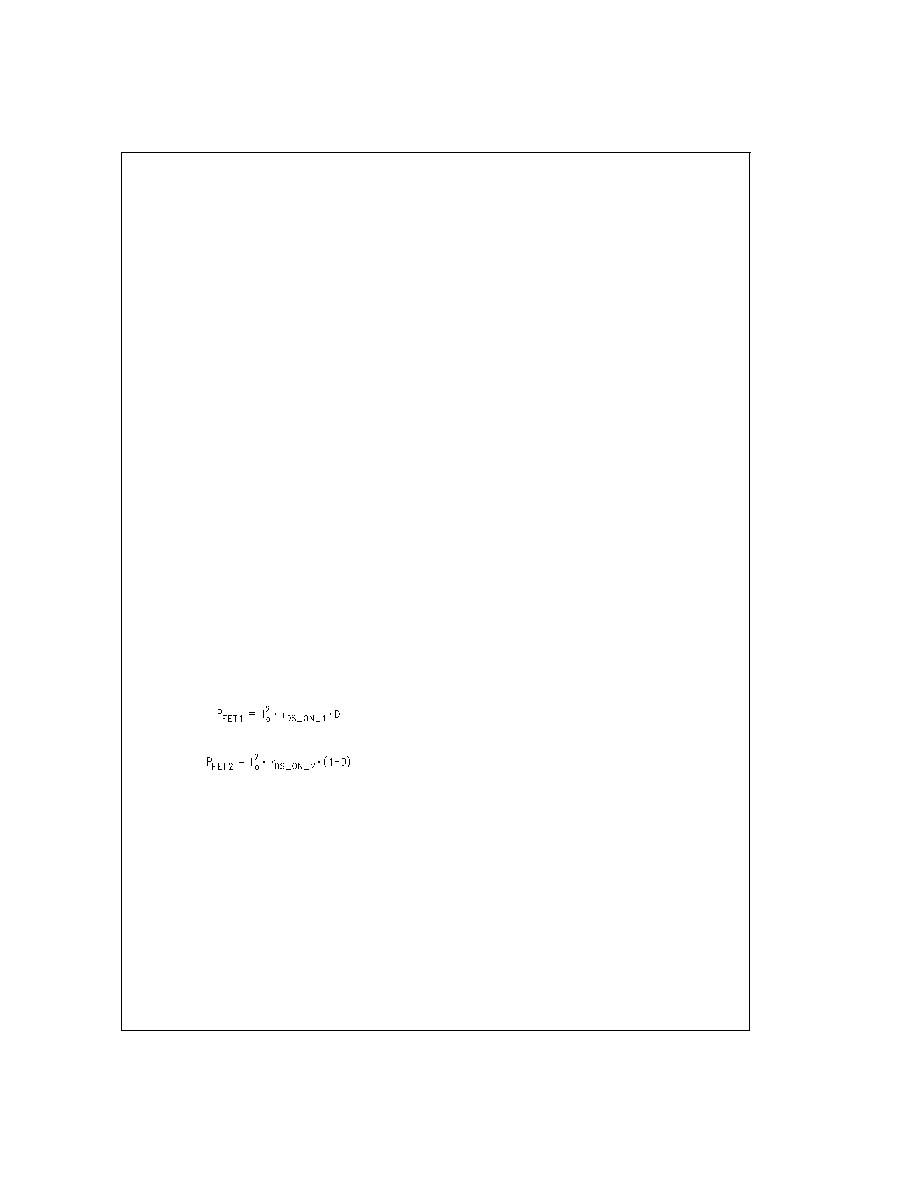
Applications Information (Continued)
FET Selection
Switching Section — The selection of FET switches affects
both the efficiency of the whole converter and the current
limit setting (if V
DS sensing mode is selected). From effi-
ciency standpoint it is suggested that for the high-side
switch, only logic level FETs be used. Standard FETs can be
used for the low-side switch when 12V is used to power the
V
DD
pin. The power loss associated with the FETs is
two-fold — Ohmic loss and switching loss. The Ohmic loss is
relatively easy to calculate whereas the switching loss is
much more difficult to estimate. The switching loss in a syn-
chronous buck converter usually happens only in the
high-side FET. When the high-side FET starts to turn on, in-
ductor current is flowing in the low-side body diode. Since
the body diode undergoes a reverse recovery before forced
off, the high-side FET will experience a pulse of drain current
turn on. The simultaneous presence of high drain-source
voltage and high drain current in the high-side FET causes
the switching loss. Apparently the switching loss is propor-
tional to the PWM frequency. Having a Schottky diode in par-
allel with the low-side body diode will to a large extent allevi-
ate the problem. This is because a Schottky diode does not
undergo a reverse recovery and it has a lower forward volt-
age than the body diode so it will take the majority of the in-
ductor current after the low-side FET is turned off. The
low-side FET benefits from what is called zero voltage
switching (ZVS). That is because every time just before the
low-side FET is turned on, inductor current is already flowing
in its body diode, resulting in a low drain-source voltage.
When the low-side FET is turned off, current will be shifted to
its body diode temporarily, again clamping the drain-source
voltage to a low value.
It is difficult to calculate the switching loss due to its compli-
cated nature. Fortunately at a reasonable PWM frequency
such as 300 kHz, the switching loss is usually much less
than the Ohmic loss. So the designer may initially ignore the
switching loss when trying to meet an efficiency specifica-
tion.
The Ohmic loss for the high-side FET is:
(12)
The Ohmic loss for the low-side FET is:
(13)
Notice when determining the r
DS_ON, the gate-source volt-
ages are usually different for the two FET’s. For the
high-side FET, V
GS is VDD minus drain voltage. For the
low-side, V
GS is VDD. This means the low-side FET may
present a lower r
DS_ON when the same type of FET is used
for both switches.
Since the r
DS_ON has a positive temperature coefficient, the
actual Ohmic loss may be somewhat higher than calculated.
The power supply designer may target 125C FET operating
temperature under maximum load and highest ambient tem-
perature and then use the corresponding r
DS_ON found in the
FET datasheet.
Linear Section — Two things need to be considered, i.e.,
r
DS_ON and thermal capacity. Make sure that the maximum
possible r
DS_ON on the N-FET is lower than the lowest
input-output differential voltage divided by maximum load
current. In a typical motherboard 3.3V to 1.5V or 3.3V to
2.5V application, this is not an issue because the maximum
allowable r
DS_ON is way higher than a typical N-FET. It is the
thermal capacity and cost that limits the selection. As an ex-
ample, consider a 3.3V to 1.5V, 4A application. The lowest
input-output differential voltage is 3.3V x 95% –1.5V x 102%
= 1.605V, so the maximum allowable r
DS_ON is 1.605V ÷ 4A
= 401 m
. Almost all low voltage discrete N-FET’s can meet
this requirement. However, the maximum power dissipation
on the FET is (3.3V x 105% –1.5V x 98%)x4A = 8W. At
least a TO-220 package with a beefy heat sink is necessary
to handle the thermal dissipation. When there is a load tran-
sient requirement such as that of the GTL+ supply, make
sure the r
DS_ON is much lower than the value calculated from
steady state operation because headroom is important for
transient performance.
Capacitor Selection
Switching Section —
Output Capacitors. The selection of capacitors is an ex-
tremely important step when designing a converter for a load
such as the MPU core. Since the typical slew rate of the load
current during a large load transient is around 20 A/s to 30
A/s, the switching converter has to rely on the output ca-
pacitors to take care of the first few microseconds. Under
such a current slew rate, ESR of the output capacitors is
more of a concern than the ESL in terms of voltage excur-
sion. Depending on the kind of capacitors being used, total
output capacitance value may or may not be an important
factor. When the output capacitance is too low, the converter
may have to have a small output inductor to quickly supply
current to the output capacitors when the load suddenly
kicks in and to quickly stop supplying current when the load
is suddenly removed. Multilayer ceramic (MLC) capacitors
can have very low ESR but also a low capacitance value
compared to other kinds of capacitors. Low ESR aluminum
electrolytic capacitors tend to have large sizes and capaci-
tance. Tantalum electrolytic capacitors can have a fairly low
ESR with a much smaller size and capacitance than the alu-
minum capacitors. Certain OSCON capacitors present ultra
low ESR and long life span. By the time the total ESR of the
output capacitor bank reaches around 9 m
, the capaci-
tance of the aluminum/tantalum/OSCON capacitors is usu-
ally already in the millifarad range. For those capacitors,
ESR is the only factor to consider. MLCs can have the same
amount of total ESR with much less capacitance, most prob-
ably under 100 F. A very small inductor, ultra fast control
loop and a high switching frequency become necessary in
such a case to deal with the fast charging/discharging rate of
the output capacitor bank.
From a cost savings standpoint, aluminum electrolytic ca-
pacitors are the most popular choice for output capacitors.
They have reasonably long life span and they tend to have
hugh capacitance to withstand the charging or discharging
process during a load transient for a fairly long period. Sanyo
MV-GX and MV-DX series’ give good performance when
enough of the capacitors are paralleled. The 6MV1500GX
capacitor has a typical ESR of 44 m
and a capacitance of
1500 F at a voltage rating of 6.3V. For a detailed procedure
for determining number of output capacitors, refer to the ap-
plication note
Using Dynamic Voltage Positioning Technique
to Reduce the Cost of Output Capacitors in Advanced Micro-
processor Power Supplies and the associated spreadsheet
for automated design.
Input Capacitors. The challenge on input capacitors is the
RMS ripple current. The large ripple current drawn by the
high-side switch tends to generate quite some heat due to
the capacitor ESR. The RMS ripple current ratings in the ca-
pacitor catalogs are usually specified under 105C. In the
www.national.com
13